Lithium from seawater was demonstrated from a research group in Saudi using a new process. This could provide nearly unlimited amounts of lithium, a critical battery material. Two new technique uses electrodes to extract lithium from seawater
Seawater could come to the rescue. The world’s oceans contain an estimated 180 billion tons of lithium. But it’s dilute, present at roughly 0.2 parts per million. Here are 2 techniques in labs that work, and 2 that don’t/
Lithium from Seaweed Using LLTO membrane
The continuous electrical pumping membrane process for seawater lithium mining has been proven at lab scale.
- The thin and dense glass-type Li0.33La0.56TiO3 (LLTO) membrane with a diameter of ∼20 mm and a thickness of ∼55 μm (LLTO) membrane provides efficient separation between lithium and other interfering ions, in addition to a high lithium permeation rate.
- The separation of the anode compartment from the feed compartment by an anion exchange membrane and the use of a saturated NaCl solution in the anode compartment allow the release of Cl2. This is necessary to prevent the dissolution of the highly soluble Cl2 in the large volume of feed stream.
- The use of a CO2 and phosphate buffer solution stabilises the pH and prolongs the lifetime of the membrane. Indeed, it was found that the LLTO membrane could be used for 2000 h with a negligible decay in performance.
- The use of a metallic copper hollow fibre enhanced the faradaic efficiency to 100% in all stages. The combination of enrichment with the conventional precipitation method make the process less sensitive to the interference of soluble ions.
The energy consumption is greatly reduced. Cost analysis showed that the value of the by-product could well overcome the energy cost
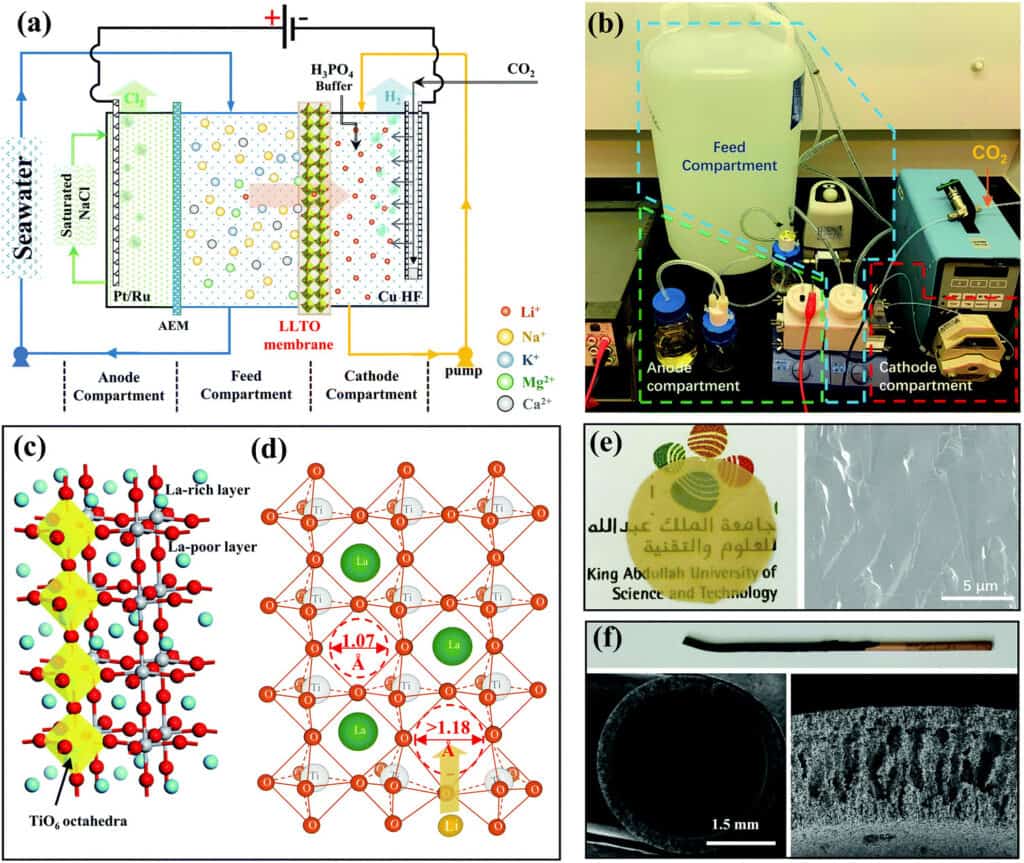
Lithium from Seawater Using Continuous Pumping With Membrane
Many researchers have tried to use lithium-ion battery electrodes to pull lithium directly from seawater and brines without the need for first evaporating the water. Those electrodes consist of sandwich-like layered materials designed to trap and hold lithium ions as a battery charges. In seawater, a negative electrical voltage applied to a lithium-grabbing electrode pulls lithium ions into the electrode. But it also pulls in sodium, a chemically similar element that is about 100,000 times more abundant in seawater than lithium. If the two elements push their way into the electrode at the same rate, sodium almost completely crowds out the lithium.
Researchers have devised numerous filters and membranes to try to selectively extract lithium from seawater. But those efforts rely on evaporating away much of the water to concentrate the lithium, which requires extensive land use and time. To date such efforts have not proved economical. But a recent paper from California suggests there may be ways to make it work.
Novel Electrochemical intercalculation
Choi’s paper reported by Science Lithium Extraction from Seawater through Pulsed Electrochemical Intercalation used a novel mechanism.
- Li extraction from seawater is demonstrated using electrochemical intercalation
- Pulsed electrochemical methods are developed to increase the selectivity of Li to Na
- 1:1 Li to Na was recovered after the electrochemical extraction from seawater
Effectively, they developed pulsed-rest and pulse-rest-reverse pulse-rest electrochemical intercalation methods with TiO2-coated FePO4 electrodes for Li extraction. The method can lower the intercalation overpotential and successfully boost the Li selectivity. Moreover, the pulse-rest-reverse pulse-rest method can also promote electrode crystal structure stability during the co-intercalation of Li and Na and prolong the lifetime of the electrode.
They demonstrated 10 cycles of successful and stable Li extraction with 1:1 of Li to Na recovery from authentic seawater, which is equivalent to the selectivity of ∼1.8 × 104. (10,000 times)/ Also, with lake water of higher initial Li/Na ratio of 1.6 × 10−3, the achieved Li extraction with more than 50:1 of Li to Na recovery.
Challenges
The authors admit though this shows great potential there are 3 issues.
- Maintainance of high faradic efficiency requires 100 to 1,000 s of electrochemical cycles before electrode replacement
- Need to develop improved hydrophilic interface coatings that will serve as a barrier against Na intrusion into the electrode.
- Fouling. Filtering of micro, nano, and molecular materials present in seawater (or alternatively salt lake water or produced water from oil recovery) would be necessary to prevent long-term fouling of the electrodes.
They conclude this is not insurmountable.
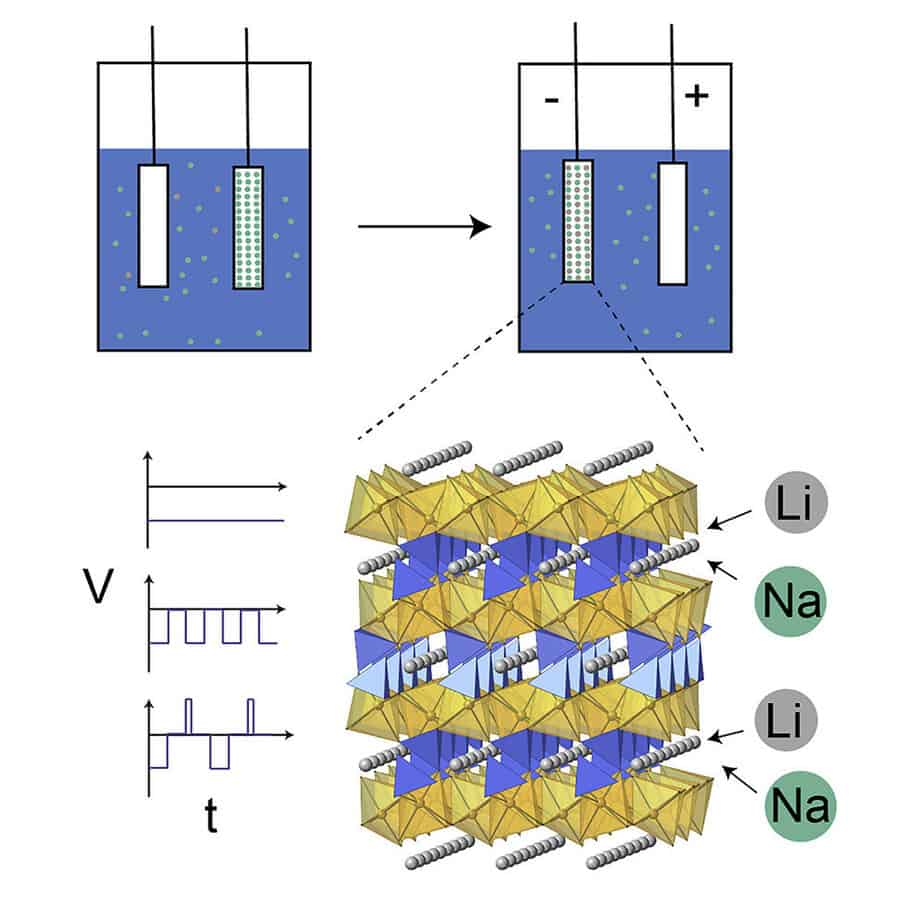
Land Resources Exhausted by 2080
Land-based resources such as salt-lake brines and high-grade ores using a chemical precipitation process are the current production method. It is technically and economically feasible only when the lithium concentration is at a level of hundreds of ppm.
Distribution of land based reserves of Lithium are both limited, and geographically uneven
- 2018, the global lithium demand reached 0.28 Mtons (Li2CO3 equivalent),
- 2020 boosted to 1.4–1.7 Mtons (Li2CO3 equivalent) by 2030
- 2080 reserves exhausted
Brine Used to Make Concrete.
Removing water in desalination creates large amounts of concentrated brine. A novel use is to use it in concrete production. Evaluation of sustainable concrete produced with desalinated reject brine
The worldwide demand for new concrete buildings is increasing at a rapid pace to keep up with urban development. Despite the need, concrete production and its use have a number of environmental consequences. The production of concrete creates a substantial need for water that directly causes a burden on the already scarce natural resource. The United Arab Emirates uses water from desalination for concrete production Desalination of seawater produces highly saline wastewater commonly known as reject brine or concentrated brine that has numerous negative environmental effects. The production of cement, the primary ingredient in the production of concrete is responsible for the generation of nearly 5% of the global carbon dioxide that is a potent greenhouse gas.
A study to reduce the carbon footprint of concrete production used reject brine as the source of water and used ground granulated blast furnace slag (GGBS) as a replacement for cement. Treatments included three different cement contents and prepared with normal tap water or reject brine. Results showed that the use of GGBS and reject brine improved the strength of concrete produced by 16.5%. Replacing 50% of the cement with GGBS and using reject brine as the source of water has a potential for reducing 176 kg CO2 and 1.7–3.4 kg of CO2 equivalents per one cubic meter of concrete, respectively. The use of the waste reject brine can potentially save USD 170–340 m3 of concrete produced.
References
- Zeng Li et al 2021 Continuous electrical pumping membrane process for seawater lithium mining Energy Environ. Sci., 2021,14, 3152-3159. DOI https://doi.org/10.1039/D1EE00354B
- Usama Ebead, Denvid Lau, Federica Lollini, Antonio Nanni, Prannoy Suraneni, Tao Yu. A review of recent advances in the science and technology of seawater-mixed concrete. Cement and Concrete Research. Volume 152, 2022, 106666, ISSN 0008-8846. https://doi.org/10.1016/j.cemconres.2021.106666.
- Kazi P. Fattah, Adil K. Al-Tamimi, Waseem Hamweyah, Fatima Iqbal,
Evaluation of sustainable concrete produced with desalinated reject brine, International Journal of Sustainable Built Environment. Volume 6, Issue 1, 2017, Pages 183-190, ISSN 2212-6090, https://doi.org/10.1016/j.ijsbe.2017.02.004. - Chong Liu Chong Liu ,Yanbin Li, Dingchang Lin, Tong Wu Yi Cui Steven Chu. 2020 Lithium Extraction from Seawater through Pulsed Electrochemical Intercalation. DOI: https://doi.org/10.1016/j.joule.2020.05.017 (https://www.cell.com/joule/fulltext/S2542-4351(20)30235-X)